What is Biological Engineering?
Biological engineers study biological processes and integrate them with engineering principles to develop solutions for a wide variety of technical problems. For example, biological engineers design medical devices ranging from glucose sensors to materials and structures for hip replacements, new processes which can clean our air and water, genetically engineer bacteria to produce fuel, pharmaceuticals, or remove toxins, and develop equipment which harvest and process our food. Biological engineers typically study many engineering disciplines such as mechanical, chemical and electrical engineering but also understand biology. This diverse expertise make biological engineers exceptionally valuable in today's challenging interdisciplinary world.
What do Biological Engineers do?
Biological engineers contribute to our society through many professional roles within academia, industry and government. Given the diversity of the biological engineering discipline, biological engineers find themselves working in a variety of fields including bioprocessing, bioenergy, environment, food production, genetic engineering, and biomedical, while always working toward a sustainable world.
Have more questions? Ask an expert!
Biological Engineers participating in the Institute of Biological Engineering (IBE) want to help you learn more about this exciting field. Click here to ask a professional Biological Engineer more.
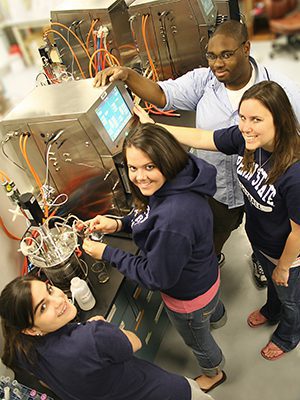
Engineering for Life
Biological engineering is the biology-based engineering discipline that integrates life sciences with engineering in the advancement and application of fundamental concepts of biological systems from molecular to ecosystem levels.
Integrating Engineering with Life Sciences
Bioprocessing/Biotechnology
Physical, chemical, and biological reactions that add value to biological systems; pharmaceuticals for humans and animals; bio-based industrial products; biodegradable products. Processing of food materials; developing and manufacturing new foods; food safety and quality control; control and movement of food materials. Production of fermented food and biochemical products. Optimizing growth and productivity of organisms. Culture and processing of aquatic plants and animals. Scale-up of biotechnology processes for new bio-based products.
Biomedicine
Designing, developing, and testing of medical equipment; medical safety; biomaterials; tissue mechanics; clinical engineering; genetic modification for bio-based pharmaceuticals/nutraceutical. Interfacing between machines and humans; human comfort and well-being. Equipment to aid the disabled; biomechanics; prosthetic devices; biosensors.
Environment
Natural resource conservation and enhancement; water quality and quantity; soil enrichment. Waste treatment; constructed wetlands; global modeling; environmental control for pets, wildlife, and zoo animals; animals as biosensors and actuators in control loops; animal health and well-being. Closed cycle and greenhouse production of plants; forest equipment and management.

About the Discipline
The Institute of Biological Engineering (IBE) was established to encourage inquiry, application and interest in biological engineering in the broadest and most liberal manner and to promote the professional development of its members.
IBE is the "headquarters" of the biological engineering discipline. IBE provides engineers, scientists and students a forum to discuss, debate and advance biological engineering.
IBE promotes the view that biological engineering is a science-based, application-independent discipline that is aligned with the perspective and foundation of biology. IBE espouses the view that biological engineers should possess the scientific knowledge of biology, including its philosophical views, be proficient in the principles and practices of engineering, and be capable of integrating discoveries from multiple disciplines to design solutions for problems.
Biological engineering is different from an application-focused engineering area like biomedical engineering, which utilizes knowledge, techniques and perspectives of electrical, mechanical, or chemical engineering and applies them to the field of medicine. As a science-based engineering discipline, biological engineering gives the flexibility to pursue any application area - from health, food and agriculture to the environment.
Biological engineering is in its nascent stage. Its body of knowledge, both in content and perspective, is at an elementary level and distinct methodologies have yet to be developed. A major focus of the biological engineering knowledge has been on the application of physical, chemical, or mathematical sciences and engineering principles to the study of biology, medicine or health. New and emerging biology-based technologies give only a glimpse of the overall potentials for biological engineering.
These perspectives simply impose the current physical sciences/engineering paradigm on biology. A deeper reflection about the biological engineering knowledge is needed. A living-system perspective, wherein decision-making is based on variable interrelationships with other living organisms, is complex and may not be amenable to reductionism. Current activities integrating biology in engineering owe their approach to the idea that in order to understand organisms or populations of organisms, one needs to understand completely their functional genomics and structural biology.
Another perspective emerges when the object-level broadens to include the scale dimensions from genetic (atomic) to organismic biology. The concept of a network of varying relationships among groups of organisms within their environment begins to appear. This shift from function to organization is represented in the shift from the mechanistic to the systemic view. The ecology of the system is modeled in terms of input/output relationships to anticipate system outcomes. Currently, the systems approach does not delve into the views from the "genetic" (atomic) approaches.
There is the question of similitude in biological engineering, that is, similarities across scales. Does the object level matter? How to incorporate holistic approaches and reductionism concurrently is a question that should be discussed. This feature is yet another attribute that distinguishes biological engineering from any of the current engineering disciplines, Biological engineering must have core "operational" concepts. At this time they appear to include transport processes at and across interfaces; locomotion; kinetics; bioenergetics; biological engineering properties of materials, products and surfaces; quantitative understanding of biology across all object-levels (from genetic to eco-level); similitude in biological engineering; and design of and within biological systems.
Major ingredients of the science-based, application-independent biological engineering discipline are core concepts based on first principles; perspectives based on living systems and uncertainty; and applications of principles of similitude in biological engineering that enables selection of appropriate object-level-based engineering approaches to problems for designing solutions.
IBE invites all those who have ideas to contribute in building the biological engineering discipline and designing our future in the new century.
Brahm P. Verma, IBE Founding President
(President 1997 and 2002)
Acknowledgement: Adaptation from the writings of Jim Dooley, Art Johnson, Joel Cuello, Brahm Verma and in the Proceedings of IBE Meetings.

Defining the Body of Knowledge
Arthur T. Johnson
University of Maryland
The plethora of new biology-based technologies has sparked a frantic rush to establish and enhance academic bioengineering programs in the U.S. The economic boom of the 1990s and the Whitaker Foundation, with its goals to contribute its entire sizeable endowment to bioengineering projects and programs by the year 2006, enabled bioengineering research and academic activity to reach an unparalleled frenzy in recent years. However, much good these pressures have been for the field, they may also have kept bioengineering from emerging in an orderly and thoughtful way necessary for the establishment of a truly independent engineering discipline.
What characterizes a discipline? There are two generally-accepted characteristics: 1) a distinct body of knowledge, and 2) distinct methods. Of the two, the body of knowledge is generally easier to define; methods require that the discipline emerges from its nascent period. Without both of these, a new discipline is just an extension of prior disciplines, and the new discipline may never achieve recognition as being separate.
There are two basic categories of engineering discipline: 1) applications-based, and 2) science-based. The first category defines the discipline by those served. For example, mining engineering, agricultural engineering, and petroleum engineering are all applications disciplines. The second category defines the discipline by its scientific foundation. For example, mechanical engineering and chemical engineering are science-based disciplines based upon mechanics (physics) and chemistry, respectively. Education in an applications discipline is usually more specific and applied than that for the fundamental science-based discipline.
It is not particularly clear whether bioengineering will become an applications discipline or a science-based discipline. Much of biomedical engineering, constituting the core of bioengineering, is applications-based. Biomedical engineering utilizes knowledge and techniques from electrical, mechanical, or chemical engineering, and applies them to the field of medicine.
Biological engineering, a term sometimes used synonymously with the term bioengineering, is science-based, and requires a strong background in biology as well as in engineering. It is recognized within biological engineering that unique solutions to technical problems may as well come from the biological side of the discipline as from the engineering side.
One big difference between these two is the loyalties, or identifications, of their practitioners. Those who practice an applications discipline are likely to have studied a more fundamental discipline and to retain their identification as part of the fundamental discipline. Thus, many biomedical engineers who work with signal processing identify themselves as electrical engineers. Many rehabilitation engineers still think of themselves as mechanical engineers. When these practitioners work with a particular application, they often do so with minimal knowledge of the system of application, and they use the methods they were taught in their fundamental discipline.
Biological engineers, on the other hand, have a thorough and intrinsic knowledge of biological systems. They are aware of nuances and expectations typical of biological systems, and identify with no other discipline.
The trouble is that the formation of biological engineering (or bioengineering) has not been completed. A body of knowledge has not been completely defined, and unique methods have not been identified. There are few true science-based bioengineers because there is no pattern for automatically forming engineers with this type of education. The students of today are being taught by the students of yesteryear, and those students were taught in fields different from biology-based biological engineering. Thus, progress toward a truly separate discipline with distinct bodies of knowledge and methods can only be incremental.
There are many distractions hindering this process of development. To use a biologically-based analogy, a new species can only emerge from isolation. Continued interbreeding with established species only adds to the richness of the gene pool, but does not lead to a new species (one trait of which is the inability to breed with other species). Likewise, the establishment of a new discipline requires a certain isolation and reflection on the vision of that discipline. In these days of unparalleled opportunity and multidisciplinary research, that isolation is not occurring. Consequently, visions for the bioengineering discipline are numerous, and often reflect the habits derived from the many source disciplines that are now feeding into what we presently call bioengineering.
Thus, we see bioengineering described in terms of biomedical engineering, which is engineering applied to medicine and health care. The biology in this vision is at the organismal level, and specifically the human organism. We also see bioengineering described in terms of cellular and tissue engineering, which is engineering applied to living cells and tissues, including genetic engineering. This vision encompasses the biotechnological and nonmedical applications, and is science-based up to the level of tissues. We also see bioengineering described in terms of biological systems engineering, which features biology and engineering as coequal foundations. This vision includes all levels of biology, including the population interactions of ecology. Because the potential applications are so numerous, this is a science-based vision.
And then there are hybrids of these approaches that combine them in different ways. The U.S. National Institutes of Health promotes one of these: by its definition bioengineering is an integration of "physical, chemical, or mathematical sciences and engineering principles for the study of biology, medicine, behavior, or health. It advances fundamental concepts, creates knowledge from the molecular to the organ systems level, and develops innovative biologics, materials, processes, implants, devices, and informatics approaches for the prevention, diagnosis, and treatment of disease, for patient rehabilitation, and for improving health."
There are opposing research directions that also influence this field. Reductionism is the tendency to view a system on its smallest possible scale. In biology, this means that to understand organisms or populations of organisms, one needs to understand completely their functional genomics. Many of the present bioengineering opportunities owe their importance to the reductionist approach.
On the other hand, other researchers are struggling to understand relations among groups of organisms and to model ecological outcomes. This holistic systems view does not presently view the system from its genetic base.
How do these two approaches affect bioengineering? They tend to pull it in two different directions. There hasn't been time nor the isolated reflection necessary to show how these approaches fit together, and, as a consequence, bioengineering is still largely undefined. At best, we can say that the field is still being formed, or in a transitional state.
The present distraction posed by cellular and tissue bioengineering is particularly strong. The opportunities are so vast that many educators have been lured into defining bioengineering in terms of their research interests. The curricula that have resulted often ignore the science of biology at levels higher than tissue. The term "bioengineering" is often applied to this portion only of the field, and this largely applications-based usage is often not distinguished from the more broadly-based scientific discipline usage of the same word that is promoted by others.
Given some thought, it could be realized that the big distinction in biology occurs at the cellular level. At or above that level, biological responses of cells or groups of cells of any complexity are fundamentally similar. Below the cellular level can be characterized as a chemical system. This discontinuity is closely analogous to that in physics above and below the atomic level. Therefore, any bioengineering that does not recognize the super cellular biological continuum is not the science-based discipline that is separate and distinct.
As far as distinctive methods are concerned, there are few candidates from which to choose. My own bias is to view the field of biology from a systems' viewpoint. Whether at a subcellular, cellular, tissue, organ, organism, or population level, biology can be considered to be another system with input/output relationships that can be anticipated as following certain general principles with some important exceptions. Looked at this way, a biological system is a product both of its genetic basis and the environment in which it finds itself. Thus, both reductionism and ecological systems approaches have their contributions to make.
It is hard to say whether bioengineering will someday emerge as a separate and distinct discipline. There are many influences from many sources that are tending to keep the field from coalescing as a cohesive unit. Until that happens, there will not be general agreement about a specific knowledge core, courses to offer, or typical academic programs to design. And, without these, industrial or other employers will not be completely sure about the capabilities of graduates from the 90 or so bioengineering programs in the U.S.
Nonetheless, these are exciting times. The opportunities are great and the contributions that an individual can make are numerous. We have a frenetic pace in the field that is not waiting for a definition or a unique discipline to emerge. That will take some time and a lot of reflective thought.
